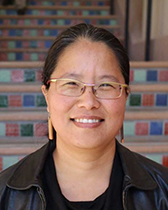
Faculty Spotlight: Songi Han
Songi Han, a Professor of Chemistry starting in Fall 2023, joins the Department from UC Santa Barbara. As part of its research program, the Han lab develops tools to push the frontiers of magnetic resonance spectroscopy and relaxometry for the study of biomolecular interactions, surfaces of biomolecular materials, and the properties of their hydration layers.
You have taken on a large challenge moving your lab across the country. What excited you to take on this challenge?
I am excited to get a go at a new start and put together a research enterprise to pursue pie-in-the-sky dreams by applying all I have learned in the past 20 years as a Professor at UC Santa Barbara now here at Northwestern University in the Chemistry Department starting on July 1. Usually in life, you do not get a chance at a do-over, you learn as you go, you stumble and fall, and make things happen by re-inventing yourself on the spot with your wit and perseverance, and that’s not necessarily a bad aspect of life. But a chance at a do-over of my life’s favorite activity (besides being a mom, ahem) of research and discovery is just amazing. When I started as a junior faculty 20 years ago, I was then also full of ideas, energy, and ambition but the ideas had not converged to a cohesive vision. I tried many different avenues and developed serval new tools that have the potential to be game changers but did not have the killer application (“the game”) ready to go yet. But hey, do we believe that the inventor of the light microscope knew ahead of time all the groundbreaking discoveries that will be enabled by the power of seeing? This is a common dilemma for tool developers. It took us many, many years to develop new tools starting from the pencil-and-paper concept, building the instrument, infrastructure, and software, to testing their implementation. Once I started the project, I did not have the luxury to pause or change my mind because that would have meant leaving an unfinished ruin. So, I had to finish what I initially envisioned to have a chance at a go. Luckily, scientific advances rely on diversity all around—diverse ideas, diverse expertise and approaches, diverse philosophies, and diverging vision, all of which requires diverse people with different background, experiences, and culture. Why culture? Because a scientist with rigorous training and a self-taught scientist with hardly any traditional training can both contribute critically and uniquely to scientific advances. You may wonder how can the absence of training be an asset. It can be because such a person must come up with out-of-the-box ideas and rely on his/her own intuition to find solutions when there is no formal theoretical framework to rely on, but I digress. My point is that tool developers who finish building their new toys out of stubbornness of finishing one’s mission and with an intuition “there must be something really cool to look at once my idea works” are as much needed as those who were driven by a clear vision to solve a scientific problem obviously critical for humanity. It turns out, there is something cool worth looking at. One can argue that every major scientific breakthrough was enabled by novel scientific tools that allowed scientists to observe nature, including us humans, in a new light, in the figurative and literal sense. Among the most exciting new tools for chemists and biologists include the technological advances in cryogenic electron microscopy (cryo-em) that allows scientists to image protein structures with atomic resolution using very sparse electrons as the “light” source. But protein structures generated by the recent resolution revolution are still static structures, like photographs of a fully functional, but idle, machine. The thing is that chemistry and biology happen in motion, in liquid water, in concert with other molecular machines, by atoms and molecules changing shapes, assembling, bumping into each other, and electrons shuffling around at the interface. Hence, it is critical to develop tools to visualize these dynamic events responsible for molecular activity and chemistry in water. The qualifier “in water” is key because processes, dynamics, and functions of molecules that make life happen are specifically tuned to work in the very special medium of water that is a liquid, and yet has intricate structures and architectures that are part of the “magic” of life. I am excited to get a reset at launching my research enterprise to focus my collective lab’s effort towards the dream of imaging molecular activities in water using technologies that I can summarily call quantum mechanical detection using magnetic spins as sensors.
If you were going to describe your research to somebody outside of chemistry, what would you tell them that it is?
The observation tools that my group is developing are aimed at capturing and detecting molecules’ shape changes, dynamics, and interactions with others in the water. The human eye lens is a specific microscopic tool that is tuned to the wavelength of visible light. The light that my lab relies on is electromagnetic waves in the radiofrequency (meter waves) to microwave frequency (cm to mm waves) range, and the length scale at which we observe nature is in the micrometer (10-6 m) down to the Angstrom (10-10 m) length scale to interrogate interactions and changes at the molecular, atomic, and electronic level. But how do we see nanometer size objects using millimeter or centimeter wavelength? We do so by relying on a fundamental property of an electron and atoms that have a magnetic property at the quantum mechanical level known as a “spin”. When we place the electron or nuclear spin in a strong magnetic field, they precess like a spinning top once it is excited (or spun) with coherent electromagnetic energies that meet the resonant condition for the spinning top (or just the right torque). The resonance condition in a high magnetic field exceeding a Tesla (1 Tesla is >20,000 fold the Earth’s magnetic field) is met with radio frequency light for nuclear spins of hydrogen, oxygen, or carbon and with microwave frequency light for electron spins of a transition metal or free radical and is the basis for nuclear magnetic resonance (NMR), magnetic resonance imaging (MRI), and electron paramagnetic resonance (EPR) spectroscopy. What my lab is doing is using electron spins as boosters to amplify the small NMR signal by orders of magnitudes, and at the same time use these electron spins as “GPS” sensors to locate the NMR signal from a specific site or the interface within 1-2 nm of the spin label GPS, and thereby turning NMR into a surface sensitive tool. This is how we detect the structure of water at molecular interfaces that are exceptionally important for the efficiency of drug formulations and the activity of surfaces but were deemed invisible. It turns out that with the right light source, at a high magnetic field, and with newly developed tools, there are ways to tease out something as “fluid” as the structure of dynamic water. However clearly, the secret sauce is the magnetic properties of electron and nuclear spins that my lab use synergistically. Water is a molecule that consists of two hydrogen atoms bonded to an oxygen atom, with an H-O-H angle near 109.4 degrees. The oxygen-17 spin resonance energy depends on the shielding of the oxygen-17 nuclear spin from the main magnetic field that is exquisitely sensitive to tiny changes in the H-O-H angle or the hydrogen bond length, i.e. the structure of water, but its detection requires an energy boost from electron spins. The magnetic coupling between water’s hydrogen-1 nuclear spin and the electron spin label depends sensitively on the speed by which water moves across the tiny magnetic field generated by the electron spins. Using this principle, my group invented an approach to measure the time it takes for water to move across 1-2 nanometers, which varies from site to site or surface to surface from tens of picoseconds to several nanoseconds and reflects on the structural property of water. The idea that water can be “structured” by molecules or ions is certainly true, but the structure of the water that you drink will immediately adjust to the physiological interfaces of the person drinking. I say, don’t waste your money purchasing a swirl structured water pitcher or magic crystal water for hundreds of dollars at Amazon.
Tell us some, tell us some of the potential applications for your research.
My group is working on uncovering fundamental mechanisms that underlie molecular motion and activities in water. These are also the mechanisms by which drugs activate brain receptors, proteins recognize friend from foe, and interfaces either activate or block chemical reactions. These key molecular events are hardly “visible” with currently available technologies. The eventual applications of a deeper understanding gained in this area are infinite. Take water at the interface of the polymer that protects the mRNA-based Covid-19 vaccine. The structure of such water can influence whether the polymer coat does its job of melting and delivering the vaccine at the right time and under the right condition. Scientists know that the structure of water in pharmaceutical formulations is what contributes to the “magic” of brand name vs generic drugs, but the property of this essential water can be considered invisible with even the most advanced tools available today. This is a key example of what my lab’s research intends to change—to observe molecular properties that are responsible for chemical and biological activity at the interaction interface.
One of our favorite problems is to study the property of tau, a protein involved in a wide range of neurodegenerative diseases that include Alzheimer’s disease and chronic traumatic encephalopathy (CTE), under simulated disease onset. Over decades, under adverse physiological conditions whose origin is unknown, tau dissociates from its native state of being bound to microtubules in the brain’s neurons and forms neurofibrillary tangles that are disease hallmarks. However, there is no physiological method of detecting tauopathies, let alone for early detection. To have a chance at developing novel approaches for the early detection of neurodegenerative diseases, we must understand the thing, the shape, that defines pathological tau in the initial stages of simulated disease onset. The challenge is that existing state-of-the-art structural biology tools are not suitable for capturing molecules such as tau that are “intrinsically disordered”, i.e. wiggly molecules that change shapes as they interact and assemble. So how do we detect the shape of tau that does not have a defined shape? We tag the protein tau with a pair of electron spin labels or a single spin label and use the intrinsic nuclear spins of the carbon of tau, the hydrogen or oxygen of water, or the carbon of tau. We use magnetic resonance tools that combine these electron and nuclear spins as boosters and spies to report on the distribution of distances of the entire spin network. The beauty of using the quantum mechanical spin at a high magnetic field is that it is a light source that never quenches or dims so that the signal is a superposition of the entire spin label ensemble. Each spin is slightly different because its energy sensitively depends on its proximity to another spin or electronic environment. Hence the superposition of signals can yield a distribution of distances that can report on the “shapeless” state of tau and/or emerging, partially folded, structures. Being able to see disordered and partially ordered structures is a critical step towards developing approaches towards early detection of pathological tau aggregation. To capture the early pathological tau aggregates the interfacial structure responsible for “infecting” naïve tau molecules to adopt the pathological shape and growth into fibrils needs to be known. We believe that the topology and structure of water at such an interface are distinct and promote nucleation and growth of tau assembly.
Our tools are geared towards detecting the structure of water at biomolecular interfaces, especially for detecting structured water that may hold the signature of a highly active surface, and is brought to “light” by detecting the magnetic shielding of oxygen-17 of water at the interface, highlighted by quantum mechanical signal transfer from site-specific spin-label to oxygen-17 to boost the tiny NMR signal of oxygen-17 with highly polarized electron spins in a process known as dynamic nuclear polarization. Our approach to developing early diagnosis for neurodegenerative requires molecular-level understanding and manipulation of interactions.
My group is also working on how to replicate nature’s success of arctic fish that use antifreeze proteins to achieve antifreeze and/or ice recrystallization inhibition. This is a critically important technology for prolonging the time window for organ donation or the preservation of cells and vaccines. How is this challenge related to our core interest? Let’s think about what a molecule must do to either prevent freezing or slow ice growth. The anti-ice molecule should bind to the ice interface while somehow hindering water molecules to approach the ice surface to grow more ice. Our hypothesis is that antifreeze molecules rearrange to have an ice-binding interface that is hydrated by structured, tetrahedral, and hence ice-like water that hence has a high affinity to the ice interface, while the interface away from the ice-binding surface is doing the job of preventing ice growth by inducing hydration with water harboring slow translational motion that prevents rapid ice nucleation and growth.
These projects have in common that developing solutions by screening for conditions or drugs without a fundamental molecular level understanding, including that of water at the interaction interface, has failed. Our hope is that our rational approach will offer new solutions to some of the important outstanding questions that may have a societal impact. Regardless, advances in our fundamental understanding of the dynamics and activity of basic building blocks of life will become inevitably critical, sooner or later.
What can students anticipate when they are working in your lab? What will the environment be like for them?
A good environment and culture in my lab are at the very core of what I define as success. My lab should be a place of learning with ambition, energy, and joy. What are the key ingredients of a good environment? My goal is to generate an environment where every student and postdoc wants to do research. You cannot make grown people (or anybody for that matter) do anything they don’t want, but I can motivate people. Because I have been in the field longer, I have a broader view, I can provide context, and I can provide a vision to help my students connect their daily actions in the lab to the bigger picture. Feeling inspired is the secret sauce to success, but how do we get there? Is it just about understanding the bigger picture? No, it is not just about knowing the bigger picture. To feel inspired, the researcher must become convinced that the vision needs him/her and develop a clear view of the concrete path that should be taken. The first step is to believe in yourself. Students come with vastly different levels of self-confidence. Some students just run with the vision and others are hesitant and unsure. Here is what I communicate to my students. Once you make it through college and come to graduate school, there is no such thing as fundamentally different capabilities. Different preparations, knowledge, and experience, yes, different confidence levels, yes, but different capabilities to do research, not really. What is relevant is, what you want to do, and do you believe in it. Research has a lot to do with character. If you are more inclined towards hardware development, that is a character thing. If you are more inclined towards biological science or hardware development, that is your preference, and that does not need to change - you play to your strengths. I tell them “It’s too hard” does not exist, “I can't do it,” or “I don't have a background” does not exist. The only relevant question is: are you interested, and do you want to do it? And if the answer is yes, I got time and patience. Happy and confident people make a good atmosphere that is key for long-term productivity. Another key ingredient for productivity is diversity. We need to have a diverse group with members who bring diverse ideas, diverse skills, and diverse perspectives and experiences that come from different backgrounds and upbringings. Researchers who witness successful projects and successful people from very different starting points and preparations will develop a deeper understanding of humanity and will make better leaders because they will learn how not to judge people by their looks if they want to assemble the strongest possible team, and that they are missing out big times if they are fooled by apparent knowledge and preparations that are good to have, but do not equate to innate capabilities that make excellent scientists. Finally, the most important operational word for me is respect in all interactions with anybody and everybody, no matter their apparent status, knowledge, preparation, and stages in their lives. That is what students can expect to have working or collaborating with my lab.
What inspired you to pursue chemistry as a career, like where? How did you get here?
It started with a good chemistry teacher in high school. I was curious and wanted to be a “discoverer” early on. I could have gone in many different other science directions, but my teacher in high school was the reason I chose to study chemistry, and this shows you again how important basic education is. Well, studying chemistry in Germany came with a wrinkle. The chemistry education in Germany is seriously synthetic chemistry oriented, so the organic and inorganic labs are very serious and intense. I was able to do it and perhaps I was even good at it, but synthesis stressed me out. Synthetic chemistry did not give me any joy because the whole time I was worried about exploding something, missing a step or just leaving out a critical ingredient without which the reaction would fail. I thought at times that studying chemistry was a mistake and thought about switching to physics, but then I discovered physical chemistry. I realize that this is a perfect way out since I still get to think about molecules and characterize them without having to synthesize them! This is an example of how the choice of research direction is a character thing. People are different. For me, equations, instruments, and complicated Hamiltonian give me joy and do not stress me out. But air-sensitive synthesis, total synthesis, or even polymer synthesis (sorry polymer chemists, but for me organic chemistry was way harder back then) had me on the toe the entire time, and I knew that this is not a thing for me. I needed to go to my equations, instrumentation, and physical intuition of chemistry. But once I discovered that there is a way to be myself, I made peace with chemistry. I love chemistry, I just needed to find the right angle.
Okay, do you have any advice for young researchers considering a career in academia?
It is important to think about whether you love research and chemistry. You must love it because an academic career is hard and long-winded with long stretches of unknown where you can easily doubt yourself. The difficulty in an academic career is there can be so many unknowns. The big challenge with academia is that you need to embrace the unknown and love the unknown. If you do, things work out, but you must believe in it. Of course, you must also push for your ideas with a really good dose of realism. To be a successful researcher, you have to stand your ground because there are so many challenges and uncertainly, rejection of grants and publications with reviews that can shake your core. Standing your ground does not mean not listening or learning, but that you are the ultimate arbiter of your research enterprise after taking all viewpoints into consideration. Despite all the challenges, I can say that I love my job!
Would you like to tell us something from your life that is not on your CV?
I have teenage children. This might sound obvious to other people, but it taught me something. Originally, I thought to some extent good parenting generates certain behavior and lifestyles. Once you go through the teenage years with your children, you realize they are truly who they are. You can support them, accept them for who they are, and guide them, but you're not going to change them. It was a big revelation to me to understand that my job is to support them and help them grow. Mental health challenges are as real and concrete as diabetes, cancer, or other debilitating conditions, and as much or as little attributable to biology, habits, or lifestyle. In the same way, you would not wing cancer with your resolve and intuition, don’t do that if you don’t feel like yourself and if life feels so heavy that it robs you of your spirit. Find a professional, shop around, and try different things, but certainly get help. Everyone should. People and parents tend to project their own experiences onto others including their children. Every child is unique, and so are students and researchers. And even if 99% of the people are one way, it means there are 1% who are different, and the 1 in 100 who is different matters as much as the other 99. Even in science, extrapolation from your observation to other systems only works in simpler systems that we have an extensive understanding of, but certainly not in people. Side note: even a cup of mineral water can harbor surprises. To see that, you must open your eyes and really look carefully with an unbiased mind. This is how we discovered in a recent paper published in 2022 in the Proceeding of the National Academy of Sciences (https://doi.org/10.1073/pnas.2206765120) that the phosphates present in bodily fluids and in everyone’s buffer solution are not simply ions, but form clusters and structures that can have important biological implications in bone formation, energy household to perhaps even memory.